Psychophysiological Methods in Neuroscience
By Zachary Infantolino and Gregory A. MillerUniversity of Delaware, University of California, Los Angeles
As a generally noninvasive subset of neuroscience methods, psychophysiological methods are used across a variety of disciplines in order to answer diverse questions about psychology, both mental events and behavior. Many different techniques are classified as psychophysiological. Each technique has its strengths and weaknesses, and knowing them allows researchers to decide what each offers for a particular question. Additionally, this knowledge allows research consumers to evaluate the meaning of the results in a particular experiment.
Learning Objectives
- Learn what qualifies as psychophysiology within the broader field of neuroscience.
- Review and compare several examples of psychophysiological methods.
- Understand advantages and disadvantages of different psychophysiological methods.
History
In the mid-19th century, a railroad worker named Phineas Gage was in charge of setting explosive charges for blasting through rock in order to prepare a path for railroad tracks. He would lay the charge in a hole drilled into the rock, place a fuse and sand on top of the charge, and pack it all down using a tamping iron (a solid iron rod approximately one yard long and a little over an inch in diameter). On a September afternoon when Gage was performing this task, his tamping iron caused a spark that set off the explosive prematurely, sending the tamping iron flying through the air.
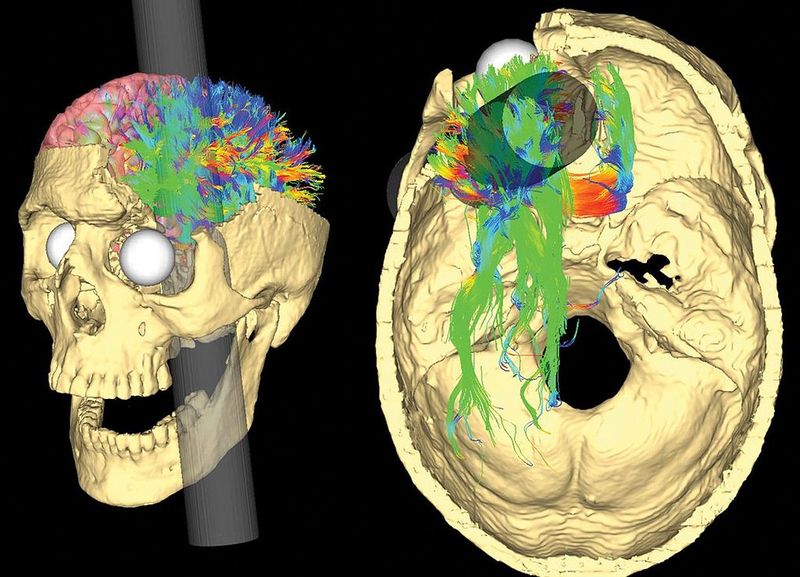
Unfortunately for Gage, his head was above the hole and the tamping iron entered the side of his face, passed behind his left eye, and exited out of the top of his head, eventually landing 80 feet away. Gage lost a portion of his left frontal lobe in the accident, but survived and lived for another 12 years. What is most interesting from a psychological perspective is that Gage’s personality changed as a result of this accident. He became more impulsive, he had trouble carrying out plans, and, at times, he engaged in vulgar profanity, which was out of character. This case study leads one to believe that there are specific areas of the brain that are associated with certain psychological phenomena. When studying psychology, the brain is indeed an interesting source of information. Although it would be impossible to replicate the type of damage done to Gage in the name of research, methods have developed over the years that are able to safely measure different aspects of nervous system activity in order to help researchers better understand psychology as well as the relationship between psychology and biology.
Introduction
Psychophysiology is defined as any research in which the dependent variable (what the researcher measures) is a physiological measure, and the independent variable (what the researcher manipulates) is behavioral or mental. In most cases the work is done noninvasively with awake human participants. Physiological measures take many forms and range from blood flow or neural activity in the brain to heart rate variability and eye movements. These measures can provide information about processes including emotion, cognition, and the interactions between them. In these ways, physiological measures offer a very flexible set of tools for researchers to answer questions about behavior, cognition, and health.
Psychophysiological methods are a subset of the very large domain of neuroscience methods. Many neuroscience methods are invasive, such as involving lesions of neural tissue, injection of neutrally active chemicals, or manipulation of neural activity via electrical stimulation. The present survey emphasizes noninvasive methods widely used with human subjects.
Crucially, in examining the relationship between physiology and overt behavior or mental events, psychophysiology does not attempt to replace the latter with the former. As an example, happiness is a state of pleasurable contentment and is associated with various physiological measures, but one would not say that those physiological measures are happiness. We can make inferences about someone’s cognitive or emotional state based on his or her self-report, physiology, or overt behavior. Sometimes our interest is primarily in inferences about internal events and sometimes primarily in the physiology itself. Psychophysiology addresses both kinds of goals.
Central Nervous System (CNS)
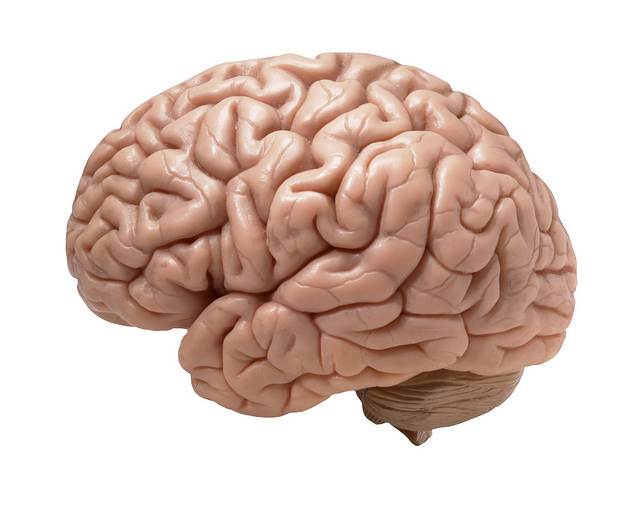
This module provides an overview of several popular psychophysiological methods, though it is far from exhaustive. Each method can draw from a broad range of data-analysis strategies to provide an even more expansive set of tools. The psychophysiological methods discussed below focus on the central nervous system. Structural magnetic resonance imaging (sMRI) is a noninvasive technique that allows researchers and clinicians to view anatomical structures within a human. The participant is placed in a magnetic field that may be 66,000 times greater than the Earth’s magnetic field, which causes a small portion of the atoms in his or her body to line up in the same direction. The body is then pulsed with low-energy radio frequencies that are absorbed by the atoms in the body, causing them to tip over. As these atoms return to their aligned state, they give off energy in the form of harmless electromagnetic radiation, which is measured by the machine. The machine then transforms the measured energy into a three-dimensional picture of the tissue within the body. In psychophysiology research, this image may be used to compare the size of structures in different groups of people (e.g., are areas associated with pleasure smaller in individuals with depression?) or to increase the accuracy of spatial locations as measured with functional magnetic resonance imaging (fMRI).
Functional magnetic resonance imaging (fMRI) is a method that is used to assess changes in activity of tissue, such as measuring changes in neural activity in different areas of the brain during thought. This technique builds on the principles of sMRI and also uses the property that, when neurons fire, they use energy, which must be replenished. Glucose and oxygen, two key components for energy production, are supplied to the brain from the blood stream as needed. Oxygen is transported through the blood using hemoglobin, which contains binding sites for oxygen. When these sites are saturated with oxygen, it is referred to as oxygenated hemoglobin. When the oxygen molecules have all been released from a hemoglobin molecule, it is known as deoxygenated hemoglobin. As a set of neurons begin firing, oxygen in the blood surrounding those neurons is consumed, leading to a reduction in oxygenated hemoglobin. The body then compensates and provides an abundance of oxygenated hemoglobin in the blood surrounding that activated neural tissue. When activity in that neural tissue declines, the level of oxygenated hemoglobin slowly returns to its original level, which typically takes several seconds.
fMRI measures the change in the concentration of oxygenated hemoglobin, which is known as the blood-oxygen-level-dependent (BOLD) signal. This leads to two important facts about fMRI. First, fMRI measures blood volume and blood flow, and from this we infer neural activity; fMRI does not measure neural activity directly. Second, fMRI data typically have poor temporal resolution (the precision of measurement with respect to time); however, when combined with sMRI, fMRI provides excellent spatial resolution (the ability to distinguish one object from another in space). Temporal resolution for fMRI is typically on the order of seconds, whereas its spatial resolution is on the order of millimeters. Under most conditions there is an inverse relationship between temporal and spatial resolution—one can increase temporal resolution at the expense of spatial resolution and vice versa.
This method is valuable for identifying specific areas of the brain that are associated with different physical or psychological tasks. Clinically, fMRI may be used prior to neurosurgery in order to identify areas that are associated with language so that the surgeon can avoid those areas during the operation. fMRI allows researchers to identify differential or convergent patterns of activation associated with tasks. For example, if participants are shown words on a screen and are expected to indicate the color of the letters, are the same brain areas recruited for this task if the words have emotional content or not? Does this relationship change in psychological disorders such as anxiety or depression? Is there a different pattern of activation even in the absence of overt performance differences? fMRI is an excellent tool for comparing brain activation in different tasks and/or populations. Figure 1 provides an example of results from fMRI analyses overlaid on an sMRI image. The blue and orange shapes represent areas with significant changes in the BOLD signal, thus changes in neural activation.
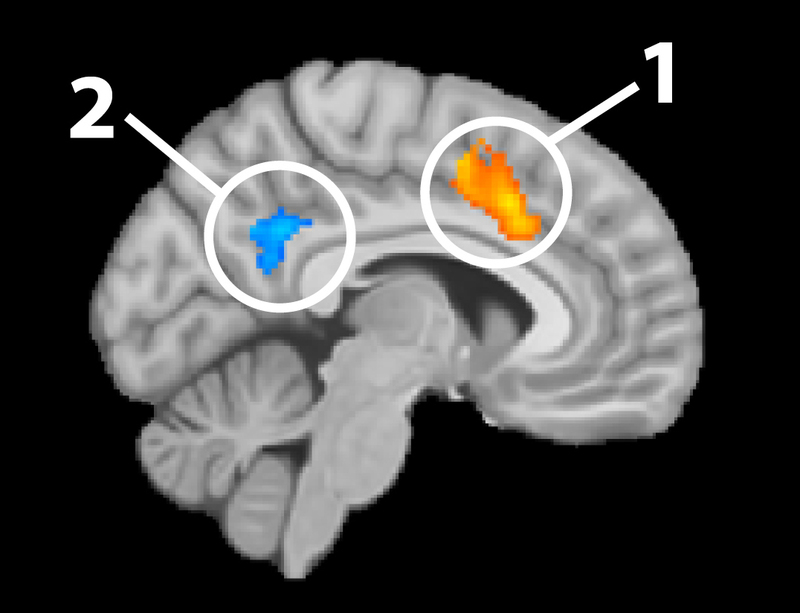
Electroencephalography (EEG) is another technique for studying brain activation. This technique uses at least two and sometimes up to 256 electrodes to measure the difference in electrical charge (the voltage) between pairs of points on the head. These electrodes are typically fastened to a flexible cap (similar to a swimming cap) that is placed on the participant’s head. From the scalp, the electrodes measure the electrical activity that is naturally occurring within the brain. They do not introduce any new electrical activity. In contrast to fMRI, EEG measures neural activity directly, rather than a correlate of that activity.
Electrodes used in EEG can also be placed within the skull, resting directly on the brain itself. This application, called electrocorticography (ECoG), is typically used prior to medical procedures for localizing activity, such as the origin of epileptic seizures. This invasive procedure allows for more precise localization of neural activity, which is essential in medical applications. However, it is generally not justifiable to open a person’s skull solely for research purposes, and instead electrodes are placed on the participant’s scalp, resulting in a noninvasive technique for measuring neural activity.
Given that this electrical activity must travel through the skull and scalp before reaching the electrodes, localization of activity is less precise when measuring from the scalp, but it can still be within several millimeters when localizing activity that is near the scalp. One major advantage of EEG is its temporal resolution. Data can be recorded thousands of times per second, allowing researchers to document events that happen in less than a millisecond. EEG analyses typically investigate the change in amplitude or frequency components of the recorded EEG on an ongoing basis or averaged over dozens of trials (see Figure 2).
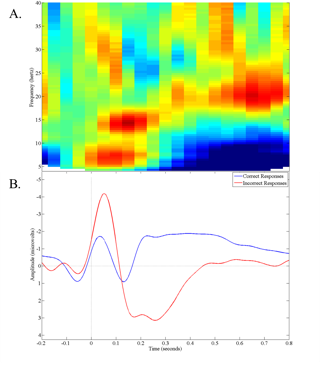
Magnetoencephalography (MEG) is another technique for noninvasively measuring neural activity. The flow of electrical charge (the current) associated with neural activity produces very weak magnetic fields that can be detected by sensors placed near the participant’s scalp. The number of sensors used varies from a few to several hundred. Due to the fact that the magnetic fields of interest are so small, special rooms that are shielded from magnetic fields in the environment are needed in order to avoid contamination of the signal being measured. MEG has the same excellent temporal resolution as EEG. Additionally, MEG is not as susceptible to distortions from the skull and scalp. Magnetic fields are able to pass through the hard and soft tissue relatively unchanged, thus providing better spatial resolution than EEG. MEG analytic strategies are nearly identical to those used in EEG. However, the MEG recording apparatus is much more expensive than EEG, so MEG is much less widely available.
EEG and MEG are both excellent for elucidating the temporal dynamics of neural processes. For example, if someone is reading a sentence that ends with an unexpected word (e.g., Michelle is going outside to water the book), how long after he or she reads the unexpected word does he or she recognize this as unexpected? In addition to these types of questions, EEG and MEG methods allow researchers to investigate the degree to which different parts of the brain “talk” to each other. This allows for a better understanding of brain networks, such as their role in different tasks and how they may function abnormally in psychopathology.
Positron emission tomography (PET) is a medical imaging technique that is used to measure processes in the body, including the brain. This method relies on a positron-emitting tracer atom that is introduced into the blood stream in a biologically active molecule, such as glucose, water, or ammonia. A positron is a particle much like an electron but with a positive charge. One example of a biologically active molecule is fludeoxyglucose, which acts similarly to glucose in the body. Fludeoxyglucose will concentrate in areas where glucose is needed—commonly areas with higher metabolic needs. Over time, this tracer molecule emits positrons, which are detected by a sensor. The spatial location of the tracer molecule in the brain can be determined based on the emitted positrons. This allows researchers to construct a three-dimensional image of the areas of the brain that have the highest metabolic needs, typically those that are most active. Images resulting from PET usually represent neural activity that has occurred over tens of minutes, which is very poor temporal resolution for some purposes. PET images are often combined with computed tomography (CT) images to improve spatial resolution, as fine as several millimeters. Tracers can also be incorporated into molecules that bind to neurotransmitter receptors, which allow researchers to answer some unique questions about the action of neurotransmitters. Unfortunately, very few research centers have the equipment required to obtain the images or the special equipment needed to create the positron-emitting tracer molecules, which typically need to be produced on site.
Transcranial magnetic stimulation (TMS) is a noninvasive method that causes depolarization or hyperpolarization in neurons near the scalp. This method is not considered psychophysiological because the independent variable is physiological, rather than the dependent. However, it does qualify as a neuroscience method because it deals with the function of the nervous system, and it can readily be combined with conventional psychophysiological methods. In TMS, a coil of wire is placed just above the participant’s scalp. When electricity flows through the coil, it produces a magnetic field. This magnetic field travels through the skull and scalp and affects neurons near the surface of the brain. When the magnetic field is rapidly turned on and off, a current is induced in the neurons, leading to depolarization or hyperpolarization, depending on the number of magnetic field pulses. Single- or paired-pulse TMS depolarizes site-specific neurons in the cortex, causing them to fire. If this method is used over primary motor cortex, it can produce or block muscle activity, such as inducing a finger twitch or preventing someone from pressing a button. If used over primary visual cortex, it can produce sensations of flashes of light or impair visual processes. This has proved to be a valuable tool in studying the function and timing of specific processes such as the recognition of visual stimuli. Repetitive TMS produces effects that last longer than the initial stimulation. Depending on the intensity, coil orientation, and frequency, neural activity in the stimulated area may be either attenuated or amplified. Used in this manner, TMS is able to explore neural plasticity, which is the ability of connections between neurons to change. This has implications for treating psychological disorders as well as understanding long-term changes in neuronal excitability.
Peripheral Nervous System
The psychophysiological methods discussed above focus on the central nervous system. Considerable research has also focused on the peripheral nervous system. These methods include skin conductance, cardiovascular responses, muscle activity, pupil diameter, eye blinks, and eye movements. Skin conductance, for example, measures the electrical conductance (the inverse of resistance) between two points on the skin, which varies with the level of moisture. Sweat glands are responsible for this moisture and are controlled by the sympathetic nervous system (SNS). Increases in skin conductance can be associated with changes in psychological activity. For example, studying skin conductance allows a researcher to investigate whether psychopaths react to fearful pictures in a normal way. Skin conductance provides relatively poor temporal resolution, with the entire response typically taking several seconds to emerge and resolve. However, it is an easy way to measure SNS response to a variety of stimuli.
Cardiovascular measures include heart rate, heart rate variability, and blood pressure. The heart is innervated by the parasympathetic nervous system (PNS) and SNS. Input from the PNS decreases heart rate and contractile strength, whereas input from the SNS increases heart rate and contractile strength. Heart rate can easily be monitored using a minimum of two electrodes and is measured by counting the number of heartbeats in a given time period, such as one minute, or by assessing the time between successive heartbeats. Psychological activity can prompt increases and decreases in heart rate, often in less than a second, making heart rate a sensitive measure of cognition. Measures of heart rate variability are concerned with consistency in the time interval between heartbeats. Changes in heart rate variability are associated with stress as well as psychiatric conditions. Figure 3 is an example of an electrocardiogram, which is used to measure heart rate and heart rate variability. These cardiovascular measures allow researchers to monitor SNS and PNS reactivity to various stimuli or situations. For example, when an arachnophobe views pictures of spiders, does their heart rate increase more than that of a person not afraid of spiders?
Electromyography (EMG) measures electrical activity produced by skeletal muscles. Similar to EEG, EMG measures the voltage between two points. This technique can be used to determine when a participant first initiates muscle activity to engage in a motor response to a stimulus or the degree to which a participant begins to engage in an incorrect response (such as pressing the wrong button), even if it is never visibly executed. It has also been used in emotion research to identify activity in muscles that are used to produce smiles and frowns. Using EMG, it is possible to detect very small facial movements that are not observable from looking at the face. The temporal resolution of EMG is similar to that of EEG and MEG.
Valuable information can also be gleaned from eye blinks, eye movements, and pupil diameter. Eye blinks are most often assessed using EMG electrodes placed just below the eyelid, but electrical activity associated directly with eye blinks or eye movements can be measured with electrodes placed on the face near the eyes, because there is voltage across the entire eyeball. Another option for the measurement of eye movement is a camera used to record video of an eye. This video method is particularly valuable when determination of absolute direction of gaze (not just change in direction of gaze) is of interest, such as when the eyes scan a picture. With the help of a calibration period in which a participant looks at multiple, known targets, eye position is then extracted from each video frame during the main task and compared with data from the calibration phase, allowing researchers to identify the sequence, direction, and duration of gaze fixations. For example, when viewing pleasant or unpleasant images, people spend different amounts of time looking at the most arousing parts. This, in turn, can vary as a function of psychopathology. Additionally, the diameter of a participant’s pupil can be measured and recorded over time from the video record. As with heart rate, pupil diameter is controlled by competing inputs from the SNS and PNS. Pupil diameter is commonly used as an index of mental effort when performing a task.
When to Use What
As the reader, you may be wondering, how do I know what tool is right for a given question? Generally, there are no definitive answers. If you wanted to know the temperature in the morning, would you check your phone? Look outside to see how warm it looks? Ask your roommate what he or she is wearing today? Look to see what other people are wearing? There is not a single way to answer the question. The same is true for research questions. However, there are some guidelines that one can consider. For example, if you are interested in what brain structures are associated with cognitive control, you wouldn’t use peripheral nervous system measures. A technique such as fMRI or PET might be more appropriate. If you are interested in how cognitive control unfolds over time, EEG or MEG would be a good choice. If you are interested in studying the bodily response to fear in different groups of people, peripheral nervous system measures might be most appropriate. The key to deciding what method is most appropriate is properly defining the question that you are trying to answer. What aspects are most interesting? Do you care about identifying the most relevant brain structures? Temporal dynamics? Bodily responses? Then, it is important to think about the strengths and weaknesses of the different psychophysiological measures and pick one, or several, whose attributes work best for the question at hand. In fact, it is common to record several at once.
Conclusion
The outline of psychophysiological methods above provides a glimpse into the exciting techniques that are available to researchers studying a broad range of topics from clinical to social to cognitive psychology. Some of the most interesting psychophysiological studies use several methods, such as in sleep assessments or multimodal neuroimaging. Psychophysiological methods have applications outside of mainstream psychology in areas where psychological phenomena are central, such as economics, health-related decision making, and brain–computer interfaces. Examples of applications for each method are provided above, but this list is by no means exhaustive. Furthermore, the field is continually evolving, with new methods and new applications being developed. The wide variety of methods and applications provide virtually limitless possibilities for researchers.
Outside Resources
- Book: Luck, S. J. (2005). An introduction to the event-related potential technique. Cambridge, MA: MIT Press.
- Book: Poldrack, R. A., Mumford, J. A., & Nichols, T. E. (2011). Handbook of functional MRI data analysis. New York: Cambridge University Press.
- Web: For visualizations on MRI physics (requires a free registration):
- http://www.imaios.com/en/e-Courses/e-MRI/NMR/
Discussion Questions
- Pick a psychological phenomenon that you would like to know more about. What specific hypothesis would you like to test? What psychophysiological methods might be appropriate for testing this hypothesis and why?
- What types of questions would require high spatial resolution in measuring brain activity? What types of questions would require high temporal resolution?
- Take the hypothesis you picked in the first question, and choose what you think would be the best psychophysiological method. What additional information could you obtain using a complementary method? For example, if you want to learn about memory, what two methods could you use that would each provide you with distinct information?
- The popular press has shown an increasing interest in findings that contain images of brains and neuroscience language. Studies have shown that people often find presentations of results that contain these features more convincing than presentations of results that do not, even if the actual results are the same. Why would images of the brain and neuroscience language be more convincing to people? Given that results with these features are more convincing, what do you think is the researcher’s responsibility in reporting results with brain images and neuroscience language?
- Many claims in the popular press attempt to reduce complex psychological phenomena to biological events. For example, you may have heard it said that schizophrenia is a brain disorder or that depression is simply a chemical imbalance. However, this type of “reductionism” so far does not appear to be tenable. There has been surprisingly little discussion of possible causal relationships, in either direction, between biological and psychological phenomena. We are aware of no such documented causal mechanisms. Do you think that it will ever be possible to explain how a change in biology can result in a change of a psychological phenomenon, or vice versa?
Vocabulary
- Blood-oxygen-level-dependent (BOLD)
- The signal typically measured in fMRI that results from changes in the ratio of oxygenated hemoglobin to deoxygenated hemoglobin in the blood.
- Central nervous system
- The part of the nervous system that consists of the brain and spinal cord.
- Deoxygenated hemoglobin
- Hemoglobin not carrying oxygen.
- Depolarization
- A change in a cell’s membrane potential, making the inside of the cell more positive and increasing the chance of an action potential.
- Hemoglobin
- The oxygen-carrying portion of a red blood cell.
- Hyperpolarization
- A change in a cell’s membrane potential, making the inside of the cell more negative and decreasing the chance of an action potential.
- Invasive Procedure
- A procedure that involves the skin being broken or an instrument or chemical being introduced into a body cavity.
- Lesions
- Abnormalities in the tissue of an organism usually caused by disease or trauma.
- Neural plasticity
- The ability of synapses and neural pathways to change over time and adapt to changes in neural process, behavior, or environment.
- Neuroscience methods
- A research method that deals with the structure or function of the nervous system and brain.
- Noninvasive procedure
- A procedure that does not require the insertion of an instrument or chemical through the skin or into a body cavity.
- Oxygenated hemoglobin
- Hemoglobin carrying oxygen.
- Parasympathetic nervous system (PNS)
- One of the two major divisions of the autonomic nervous system, responsible for stimulation of “rest and digest” activities.
- Peripheral nervous system
- The part of the nervous system that is outside the brain and spinal cord.
- Positron
- A particle having the same mass and numerically equal but positive charge as an electron.
- Psychophysiological methods
- Any research method in which the dependent variable is a physiological measure and the independent variable is behavioral or mental (such as memory).
- Spatial resolution
- The degree to which one can separate a single object in space from another.
- Sympathetic nervous system (SNS)
- One of the two major divisions of the autonomic nervous system, responsible for stimulation of “fight or flight” activities.
- Temporal resolution
- The degree to which one can separate a single point in time from another.
- Voltage
- The difference in electric charge between two points.
Authors
- Zachary InfantolinoZach Infantolino, Clinical Psychology Graduate Student at the University of Delaware, studies the neural mechanisms associated with motivation and executive functions and how they are impacted by anxiety and depression. He is involved in treating individuals suffering from anxiety and depression, using his research and clinical work to inform one another.
- Gregory A. MillerGregory A. Miller is Professor and Chair of the Department of Psychology at the University of Delaware and Professor Emeritus at the University of Illinois at Urbana-Champaign. He has served as Director of the Biomedical Imaging Center at Illinois and as President of the Society for Psychophysiological Research.
Creative Commons License



